Nuclear Thermal Propulsion
Introduction
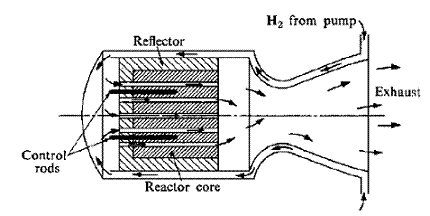
Nuclear thermal propulsion concept
Nuclear thermal propulsion is conceptually similar to solar thermal propulsion except for the source of heat. In nuclear thermal propulsion, the heat released from nuclear fission is used to burn the propellant.
Concept
At the fundamental level, all nuclear fission reactors convert a nuclear mass m into energy E according to E = mc², where c is the speed of light. Fission is the process in which neutrons are absorbed by the fuel material. A fissile fuel, usually uranium or plutonium, converts a percentage of its mass into energy when its nuclei are split by neutrons. The excitement of the fuel atoms produces thermal energy which is then used to heat the propellant. The heated propellant flows through the core of a nuclear reactor, and expands through a rocket nozzle to create thrust. To produce greater impulse and efficiency, higher temperatures in the reactor core are needed. In addition, a low propellant molecular mass will lead to a greater expansion of the propellant gas which in turn generates greater nozzle pressure. As such, hydrogen is mainly used as the propellant. Effectively, a nuclear thermal propulsion system can produce 107 times greater energy density than a chemical propulsion system.
Classification: Solid Core, Liquid Core and Gas Core
There are 3 different types of nuclear thermal propulsion. They are solid core, liquid core and gas core.
Solid core is arguably the most conventional due to its ease of implementation. Evidently, the limitations of such cores lie in the melting point of the material used to construct them. At the present moment, there are no materials known to us that can withstand the heat generated by the fuel operating at maximum capability. As such, such cores can only be expected to produce impulses of up to 900 s.
A liquid-core engine which involves a rotating solid cylinder can be used to contain the fuel at a higher temperature. The induced centripetal force causes the fuel, which is of a higher molecular mass than the propellant, to the cylindrical wall. As the fuel melts and rises to temperatures above the melting point of the cylinder, the inner cylindrical wall naturally melts. The twist to this engine lies in the centripetal force which keeps the molten layer intact. Additionally, coolants running on the outside of the cylinder ensure that the entire cylinder does not melt through. The fuel is therefore able to be brought to a higher temperature than that in a solid core, and the propellant expelled with a greater force. Liquid-core engines can attain a much higher specific impulse of 1600 s.
The gas-core engine is a variation of the liquid-core design. Gaseous uranium fuel is produced in the centre of the reactor surrounded by hydrogen. This is caused by the rapid circulation of the fluid. The temperature of the reactor core could reach tens of thousands of degrees because the fuel does not come in contact with the walls of the reactor.
Performance
Nuclear thermal propulsion provides a greater specific impulse as compared to chemical propulsion. Specific impulses of 800 s obtainable by nuclear thermal propulsion utilising a solid core are twice that achievable by its chemical counterpart. The greater specific impulse and lower propellant molecular weight increase the propulsive force per unit propellant flow. As the same performance is obtainable for a reduced propellant mass, a greater payload mass can be delivered into space with a nuclear thermal system. A spacecraft would then be able to attain faster transfers of orbit which minimises travel time to the destination.
Nuclear thermal engines are not designed to accelerate payloads into space but to function in the vacuum of space. Therefore addition shielding is required to prevent radiation from scattering off the atmosphere and back onto the payload, which will hinder the proper operation of the engine during launch.
In addition, in the event of atmospheric or orbital rocket failure, there will inevitably be the release of hazardous radioactive materials into the environment. However, the environmental risks are modest. It will take more than a hundred launch failures to cause the same amount of fission activity as a sunken nuclear submarine.
Applications
In 1955, Project Rover was started at Los Alamos National Laboratory. The purpose was to develop a solid-core nuclear thermal propulsion rocket using liquid hydrogen as the propellant. A variety of systems were then developed. One of them has the capability to provide 200,000 pounds of thrust while another can produce a specific impulse of 845 s.
In 1963, an American rocket program, NERVA, was initiated to conduct experiments on the usage of nuclear thermal propulsion for long-range manned space missions. The purpose was to build a functioning rocket engine based on the graphite-based nuclear reactor that was built during the Rover project. The project was remarkably successful and it tested the practical operational capability of the nuclear thermal rocket.
However, the programs were halted in 1973 due to a combination of political, technical and budget reasons.
Future Developments
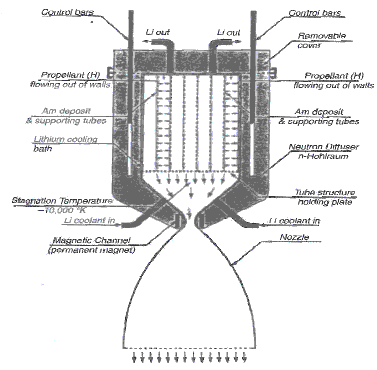
Schematic view of Rubbia’s engine
In the 1990s, Nobel Prize winner Carlo Rubbia discovered a rare isotope of the transuranic element Americium (Am-242m). The comparative advantage of Am-242m over isotopes of uranium or plutonium lies in its chemical property. Due to its lower critical mass, it can be produced in the form of thin sheets of less than 1 micron thickness. This enables fission products to ionise and escape the fuel element easily. It has also been proven that it only takes the fission of a small quantity of Am-242m to produce a huge amount of energy. Such ability to pack a big punch in a light and compact fuel allows for a smaller and lighter nuclear generator. Utilising such a generator in nuclear thermal propulsion will allow for greater weight allocation to the payload, significantly improving the efficiency. The impulses of such rockets have been calculated to be at an amazing 2000–4000 s, taking man to Mars in just 2 weeks!
In 1999, Rubbia proposed to have Am-242m implemented into a space rocket to heat the rocket propellant. Such a rocket would have the capacity for a full-size interplanetary mission. This engine may be conceivable within the next 20 or 30 years.
Comparison with Conventional Rocket Drives
Nuclear thermal propulsion is more advantageous over conventional systems in terms of propulsive power because nuclear reactions can produce large quantities of energy from little material mass.
The table below shows comparisons between conventional chemical propulsion, nuclear thermal propulsion and the future Rubbia’s engine.
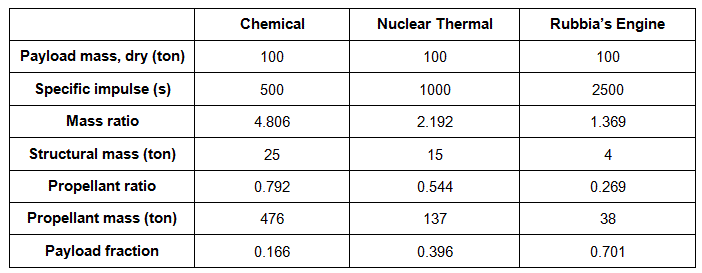
Comparison between conventional chemical, nuclear thermal and Rubbia engines
Conclusion
Comparatively, nuclear thermal propulsion holds a plethora of advantages over conventional drives. Higher impulses, lower potential thrusts amongst others will make this propulsion a much more attractive option than conventional ones. Its thrust density is low too, gifting it with an ease of lift to Earth’s orbit, a trait which few other unconventional rocket drives possess. The best of it all is that there are no fundamental technological obstacles to the construction of a nuclear thermally propelled spacecraft.
However, due to a slew of political and budget issues in the 70s, space agencies have since shelved any development plans for this propulsion. Such aversion to nuclear propulsion will hopefully melt away following the discovery of Americium. The Rubbia engine has since been proven to be mission-capable. It is our hope that this new technology will revive the spark in nuclear thermal propulsion and afford it the justification it deserves.
Bibliography
- Accettura, A., and Ferretti, A., "PROPULSION 2000 PROGRAM, Phrase 1," Final Rep., AVIO, Colleferro (Rome), Italy, NTEPRP 10000, Nov. 2000
- Claudio Bruno. "Advanced Propulsion Systems and Technologies. Today to 2020", American Institute of Aeronautics and Astronautics, Inc, 2008
- Darwar, J. A., To the End of the Solar System: The Story of the Nuclear Rocket, The Univ. Press of Kentucky, Lexington, KY, 2004.
- "Nuclear Thermal Rocket," Wikipedia, <http://en.wikipedia.org/wiki/Nuclear_thermal_rocket>
- "Nuclear Propulsion," Mars Academy, <http://library.thinkquest.org/12145/propul5.htm>
- Milton Klein, "Nuclear Thermal Rocket—An Established Space Propulsion Technology" <http://scitation.aip.org/getabs/servlet/GetabsServlet?prog=normal&id=APCPCS000699000001000413000001&idtype=cvips&gifs=yes>
- Steven D. Howe, "Assessment of the Advantages and the Feasibility of a Nuclear Rocket for a Manned Mars Mission," June 1985
- Jay W. Kelley, "Unconventional Spacelift," February, 1994
- S. Howe, S. Borowski, C. Motloch, I. Helms, N. Diaz, S. Anghaie and T. Latham "Innovative Nuclear Thermal Propulsion Technology Evaluation" 17 Oct 1991