Solar Sails
Introduction
Back in the 1600s, a physicist Johannes Kepler postulated the presence of solar breeze from his observations of comet tails. That led to the formulation of a miraculous method of space travel that does not require fuel and is capable of lasting forever. However it was not until the 1970s that the construction of a solar sail at the Jet Propulsion Laboratory (JPL) actually took place for a rendezvous with comet Halley. While the mission never did take place, the designs conceptualised from this mission laid the foundation for further research.
Concept of Solar Sails
Solar sails are born from the concept that light can generate force to propel spacecraft. Light consists of packets of photons which carry momentum. Photons impinging on the solar sails will undergo reflection, and a resultant force causes the solar sail to move. At a distance of 1 AU (astronomical unit), the photons exert a force of 9 N/km², or roughly one thousandth of the weight of two paper clips at the earth's surface. This force then grows exponentially with distance to produce a large impulse. Solar sails employ a technique known as tacking to control their orbits. The force exerted by sunlight on a solar sail comprises of the forces from the incoming and the reflected sunlight which are at right angles to each other. Together, they produce a net force vector that always points away from the sun. Should this force be angled against the direction of travel, the orbit will shrink towards the sun. To expand the orbit, the force should be angled in the direction of travel.
Design Parameters of Solar Sails
The characteristic acceleration a0 of solar sails is perhaps the most crucial design consideration of all. This is defined as the acceleration of a sail at 1 AU oriented normal to the sun line. The equation describing that is given as follows:
a0 = 2ηP/σ
where a0 refers to the characteristic acceleration, P the solar radiation pressure at 4.57×10-6 Pa, η the sail efficiency and σ = (ms + mp)/A which is the total mass of the sail and mass of payload per unit area of sail.
For a fixed sail area and efficiency, surfaces of characteristic acceleration can be generated. The JPL Comet Halley sail in 1977 achieved an a0 of 1 mm/s² with a sail of 800×800 m. However, to obtain high a0 of 5–6 mm/s², we must ensure that both the mass of sail and payload are light. With a fixed sail mass per unit area, we would need a corresponding increase in sail area for any increase in sail mass to maintain a fixed a0.
Material Used
The average areal density (AAD) of a solar sail is often used as the parameter for comparison purposes between materials for solar sails. It is defined as the ratio of the total weight of the solar sail to the surface area of the sail membrane. For far-term missions, a value of 1 g/m² is required. Recent laboratory tests have achieved that value with plasma etched Kapton. Present-day technology for the fabrication of thin polymeric membranes (1 to 10 μm) are only capable of building aluminised Kapton films of AAD 12 g/m². Mylar sail material could reduce it down to 7 g/m². As for the structural considerations, the booms and masts should too be made up of lightweight materials. Carbon nanotubes currently represent the best option for them. There is also ongoing research on molecular manufacturing techniques to create hyper-light and strong sail materials with mesh weaves of nanotubes less than half the wavelength of light. If manufactured it could produce sails of ADD less than 0.1 g/m², 50 times smaller than that of a Mylar sail.
Types of Solar Sails
When thin solar sails are deployed without any support, they will collapse under the bombardment of photons and flow around the payload. Stabilizing structures are therefore important. Two such stabilizing structures will be discussed here.
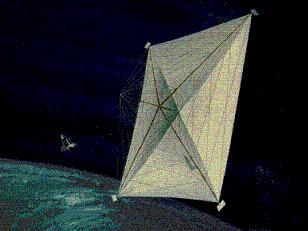
3-axis stabilised sail (JPL)
The three-axis stabilised structure, so called because it supports the sail in all three dimensions, holds the sail rigidly to catch sunlight. The outer edges of the sail are attached to booms to prevent collapse in the plane of the sail. To prevent the sail from folding up, a combination of booms, masts and stays are used. This structure allows the booms to be made lighter as the overall structure offers much more support and stability as compared to one just consisting of booms. However, these booms comprise a significant mass fraction of the entire solar sail and thus compromise on efficiency.
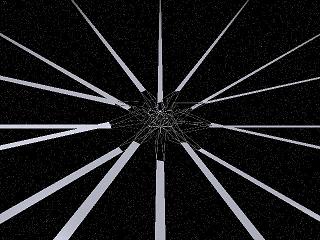
Heliogyro sail
The alternative to that would be that of spin-induced tension. The spins will stabilise the sails through centripetal acceleration. The tension lines will stretch the sails and keep it taut. Such a technique is sometimes preferred as it does away with the need to use physical materials to support the sails. The heligyro design designed by the JPL for the rendezvous with Hally's comet consists of a central hub and twelve vanes extending from it. Upon deployment, the vanes will be rolled out and kept extended via centripetal force. Ease of packing and deployment would be the principal advantage of heliogyro blades over the three-axis stabilised structure.
Laser Power Systems
Power beams from lasers have the potential to send high energy densities far out into space. For interstellar missions, usage of such concentrated electromagnetic radiation to augment the sunlight would prove immensely helpful. It would possibly reduce travel time to Alpha Centauri from 10,000 years to less than 100 years. However, the technical difficulties and the costs associated are presently insurmountable by us. When the technology becomes available, a system of laser and maser will be built to create high quality beams from a remote location in the solar system. This system will have its lenses focused on the sailing ship. With the lenses maintaining a constant power on the sail over a distance d, the velocity v that can be achieved is given by:
v² = 4dPη/cM
where M is the spacecraft mass, c speed of light, P power level and η efficiency. The energy in the output beam is in the order of hundreds of terawatts. This contrasts with our current planet power consumption of a mere 15 terawatts.
Applications
The applications of solar sails are far-reaching in the truest sense of the word. Not only would solar sails offer the usual services of interplanetary travel, they could potentially open the gateway to interstellar travel. In the most basic application, solar sails could assist a spacecraft in trajectory corrections. By tilting the solar sails in the correct orientation, a solar sail equipped spaceship could alter its course more delicately than with thrusters. The Messenger probe that has reached Mercury utilizes this feature on board. In addition, with the right tilt of the solar sails, energy from photons could be harnessed to help counteract forces of gravity, allowing a spacecraft to hover as a satellite above a planet.
Comparison with Conventional Rockets
Solar sails make for a strong case. They do not require fuel, are cost efficient, and have the theoretical ability to travel forever. Take an example of a mission that will elucidate the cost efficiency offered by solar sails over conventional rockets. For conventional rockets carrying a 20 ton payload to Mars, a 100 ton launcher vehicle will have to be developed, at a cost of roughly $10 billion. With a low launch rate, there will be high operating costs and a high amortised development cost. An additional $1 billion, inclusive of the launch and orbital rocket costs will be incurred.
In contrast, the launch of a sailing ship by the Titan 4 launch vehicle will cost a mere $150 million. Its development costs will be amortised over the numerous voyages to Mars and the number of ships produced. An estimate cost of sending a 20 ton module to Mars via sails will be at $200 million, 2% that of conventional rockets.
Future Developments
Solar sailing is not a new concept and it has gone through detailed engineering design through the years. Much of the future developments hinges on producing lighter and better structures and sails for the craft. It is a pity that till date, not a single launch of any solar sail has been successful. Cosmos 1 by The Planetary Society and NanoSail-D by NASA were just two of the several failed launches. However, there are many more in the pipeline. Should Lightsail-1, the successor of Cosmos 1 be successfully launched in the next few years, it will serve as a precursor to a whole new mode of space travel.
Bibliography
- "Setting Sails for the Stars" Science@NASA, 28 June 2000 <http://science.msfc.nasa.gov/headlines/y2000/ast28jun_1m.htm>
- Benjamin Diedrich, "Solar Sails" Caltech University, 10 April 2001 <http://www.ugcs.caltech.edu/~diedrich/solarsails.htm>
- Steve Nerlich "Cosmos 1" Cheap Astronomy <http://www.cheapastro.com/podcasts/Transcript_Cosmos1.pdf>
- Paul Glister "Solar Sails: The Interstellar Prospect" Centauri Dreams, 4 Sept 2008 <http://www.centauri-dreams.org/?p=2943>
- "Solar Sails" Wikipedia <http://en.wikipedia.org/wiki/Solar_sail>
- "World energy resources and consumption" Wikipedia <http://en.wikipedia.org/wiki/World_energy_resources_and_consumption>
- Dr Colin R McInnes Solar Sailing: technology, dynamics and mission applications, Springer, 2004
- David L. Edwards, Mircea Chipara "Polymeric materials for solar sail" Materials Research Society Symposium Preceedings (Dec 2004) volume 851
- Louis Friedman. Starsailing : Solar Sails and Interstellar Travel Wiley Science Editions, 1988
- Jerome L. Wright Space Sailing Gordon And Breach Science Publishers, 1992
- McInnes, Colin R. "Solar Sail Spacecraft." Interdisciplinary Science Reviews (Dec 1995): 289-301.